by IMEC
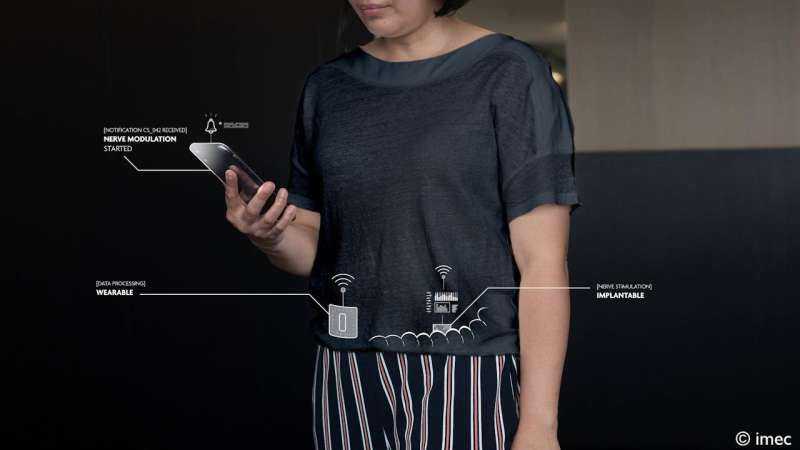
Bio-electronic medicine is a new form of therapy that uses electrical pulses to modulate nerve activity. The small implant delivering the pulses sits in the body, a wearable device delivers power to the implant and a mobile unit can be used to tweak the stimulation parameters. Credit: IMEC
The central (CNS) and peripheral (PNS) nervous system work together to coordinate and regulate the functions of our body in addition to hosting our human intelligence. The CNS is the control center and includes the brain and the spinal cord. While the brain processes information, makes decisions, and sends out instructions, the spinal cord and the associated PNS is the communication highway that carries nerve signals to and from the brain.
The PNS consists of a vast network of nerves that branch out from the brain and spinal cord to our organs, limbs, and tissue. By relaying signals, the PNS and the brain provide communication between the CNS and the rest of the body. The PNS may not have the same rock star reputation as the brain, but it is the backbone of our daily lives. It plays a pivotal role in our sensory experiences, reflexes, and even autonomic functions such as digestion and heartbeat.
Because of all these critical functions, diseases affecting the PNS can significantly impact daily life by causing limitations in mobility, sensation, and coordination. They disrupt communication in specific types of nerves, such as motor nerves responsible for muscle control, sensory nerves responsible for transmitting sensations of touch, temperature, and pain sensations, or autonomic nerves that regulate the balance in our cardiac, pulmonary and inflammatory response.
New medicinal solutions are normally developed to treat a very specific condition and the development of a new drug typically takes years to go through all the stages before it is approved and brought to market. Enter bioelectronic medicine, a novel approach that harnesses the language of electrical pulses to speak directly to the intricate network of nerves in the PNS.
Bio-electronic medicine: Electricity as a therapy
Bioelectronic medicine stimulates electrically active tissue such as nerves or muscles to suppress undesired conditions in the body, or to facilitate desired conditions. The best known example already on the market is the cardiac pacemaker, which stimulates the heart muscle. The peripheral nervous system is of particular interest in modern research on bioelectronic medicine because it is the bidirectional highway that carries signals from the brain to the organs and vice versa. By stimulating the PNS, both the organs and the brain can be addressed.
One of the advantages is that the therapy can be controlled by smart electronics: instrumentation that are adaptive and can be tuned by a doctor or even the patient after implantation. It can be switched on and off instantly with a remote control, which is not possible with orally administered drugs. In addition, the treatment can be automatically adjusted based on environmental factors or internal markers that reflect a person’s health status. Today, a number of chronic diseases such as rheumatoid arthritis, chronic pain and epilepsy are targets for this therapy.
Imec employs nanotechnology to develop small, low-power stimulating implants for various PNS applications, similar to its miniature brain implants for sensing. In fact, both brain and PNS implants share similar future goals.
First, devices for both targets are exploring solutions for increasingly detailed sensing and/or stimulation. Second, new encapsulation methods based on extensive materials research will allow the implants to function optimally in the body for months and years. Third, they both aim to combine electrical stimulation and readout in real time to eventually close the loop: stimulation in response to sensed information. Finally, researchers are looking at combining the implant technology with different modalities, such as ultrasound, for stimulation purposes but also for energy transfer to the implant.
From pills to pulses: Electrifying medicine using the peripheral nervous system(Left) Schematic cross-section of the encapsulated chip. The alternating polyimide films and ALD layers were etched in different stages, each with an adjusted masking layout, resulting in a stepped profile. This guaranteed that the side walls of the vias are always covered with a bidirectional diffusion barrier. (Right) Scanning electron microscope (SEM) image of a via towards a recording electrode that shows the stepped profile. Credit: IMEC
The vagus nerve as the ultimate target
From the spinal cord, nerves extend outward to their destination. The lower in the body, the more specific the nerves are. Stimulation at the endpoint of these nerves selectively affects the target organ, making it technologically easier to direct the electrical pulses. The flip side of the coin, however, is that the device needs to be smaller and sit deeper in the body, which poses a challenge for surgery, packaging, communication, and energy delivery.
The closer the nerves are to the brain, the more complex they are. The vagus nerve, for example, is the longest cranial nerve in the body and is directly connected to multiple organs and systems such as the heart, lungs and digestive system. With a PNS implant, stimulation of the vagus nerve can potentially affect a wide range of bodily functions from a single (and more superficial) point of intervention.
Vagus nerve stimulation in the neck has already gained acceptance for certain forms of treatment-resistant epilepsy and depression, while chronic migraine comes up as a relatively new application. But we’re not there yet. The vagus nerve is a 4-mm-wide puzzle of nerves containing subbundles (fascicles) with many different types of fibers that branch, merge and twist along the nerve. This makes stimulation of the correct fibers in the nerve essential.
For example, some epilepsy patients who have undergone this type of treatment, for example, complain of voice changes, heart palpitations or changes in breathing, because the pulse that silences the seizures also affects the fibers that run to the larynx, heart, and lungs.
Targeted stimulating translates as focusing the stimulation energy on a smaller physical unit, from millimeters to tens of millimeters. Researchers at imec and the Feinstein Institute of Medical Research are collaborating to radically improve the selectivity of nerve stimulation using a new technique called i2CS, based on interference of electric fields of different wavelengths. The main technological challenges include synchronous delivery of interferential currents through pairs of electrode and minimizing signal distortion and leakage when injecting high currents at high frequencies.
Advanced packaging for implants
Another challenge is to design a package that allows the device to remain in the body for months or years. Proper encapsulation and wiring is of primordial importance for functionality, longevity and stability. The protective barrier works both ways. On the one hand, the device should be protected from the harsh body environment as fluids can compromise its functionality. On the other hand, the device should not induce any unwanted response of the surrounding body tissue by leaking non-biocompatible materials.
The majority of FDA-approved electronic implants have a rather bulky and rigid titanium housing as a hermetic encapsulation. While a proofed concept, such an approach needs an extensive review for future implants that aim for miniaturization -both in terms of materials and packaging methods. CMST, an imec research group at the University of Ghent, develops dedicated packaging for long-term implants.
From pills to pulses: Electrifying medicine using the peripheral nervous system(Left) The chip’s layout and (Right) diagram with a system that compresses data a hundredfold by only transmitting event signatures. The resulting system includes an analog-to-spike converter which reports the events (“delta encoding”) when they cross a threshold, a spiking neural network or AI for local computation and a pulse-based transmitter tailored for low-energy event-driven transmission. Credit: IMEC
In a 2019 publication, they presented a processing platform for encapsulating a PNS implant based on thin-film manufacturing techniques. The researchers worked with soft materials instead of the traditional harder materials such as titanium (alloys) to enable miniaturization and ultra-compliance of the final device. In addition, soft encapsulation increases comfort, has a limited foreign-body response and offers the conformality needed to cover the chip topography.
Polymers, such as polyimide, have been widely used to encapsulate implantable electronic devices. However, polymer packaging suffers from moisture diffusion, swelling, poor adhesion and/or material degradation, ultimately leading to device failure. In contrast, metal oxides such as Al2O3, HfO2 and TiO2, deposited by atomic layer deposition (ALD) are ultrathin (thickness in the order of a few nanometers), extremely dense, and conformal coatings, reportedly with excellent moisture barrier properties.
To benefit from the advantages of the polyimide and the ALD layers, the researchers combined materials of both types to obtain mechanically flexible encapsulations with excellent barrier properties. They developed multilayer stacks of polyimide films with a thickness of 5.5 μm and HfO2/Al2O3/HfO2 layers with thicknesses in the range of 10 nm to 20 nm with special attention to the adhesion between the layers to avoid moisture accumulation at the interface.
This golden combination passed all common barrier tests. As an added benefit, all of the required process steps are compatible with thin-film manufacturing techniques, making the process scalable to high-volume, low-cost manufacturing.
Closing the loop
Most implants are still feed-forward: you switch the device on/off, stimulation starts/stops. The physician can toggle the intensity of the stimulation, and that’s it. One example is the device imec developed with its partner Neurogyn, for stimulation of the pelvic nerve which runs through the area around the bladder and the genitals. Stimulation calms an overactive bladder in patients with irritable bladder syndrome, but it also helps with conditions such as incontinence and certain sexual disorders. The resulting prototype is currently in the preclinical phase of medical device development.
The future, however, lies in a closed-loop system in which stimulation occurs based on measured parameters. That’s the idea behind a new project for Horizon Europe, where imec and the consortium it is part of have recently started working on an implant for an amputee’s hand stub that reads out and stimulates the arm nerve in order to move an artificial hand. For such a design, imec will draw on its expertise in signal acquisition, processing and stimulation, as well as on its experience in miniature, low-power designs with edge computing.
In order to target the right nerve, the devices have to be placed very close to the surface, for example, as a cuff or inside the nerve. These spatial constraints, in turn, limit the volume and energy of the device. The nerve implant ideally has a millimeter-scale volume and—since there’s no room for a battery—the electronic system should consume low power (well below 100 µW), to enable wireless power transfer. On the other hand, nerve signals (called spikes or action potentials) are typically sparse but very fast events (milliseconds), so a high temporal resolution of the recording system is essential. If these signals were sampled with conventional high sampling rates, there would be a high signal redundancy and thus a poor system efficiency.
The total energy consumption of information processing and transport can be significantly reduced if only the changes (i.e., delta) of the signals are processed. A team in imec The Netherlands recently translated this idea into a chip that—instead of continuously processing signals—is only active when an event occurs, i.e., up and down phases of action potentials, thereby creating temporal signatures.
Imagine that the transmitted temporal signatures could then be decoded and fed back into the implant stimulator to perform real-time closed-loop neuromodulation. This would significantly reduce control latency and the data rate, thereby reducing the wireless communication requirements as well as the power consumption.
How to power the implant
With the increasing complexity of the implants, energy-efficient powering is more important than ever. The choice of power source depends on the user scenario and is often a balance between how often the user needs the implant, how much energy it consumes, and how life-threatening the condition is it alleviates.
For example, the artificial hand from the EU project mentioned above can be removed while sleeping and charged on a bedside table. In this case, no battery or a very small implantable battery is sufficient. On the other end of the spectrum are implants that stimulate continuously, such as the Neurogyn stimulator for an overactive bladder. These require larger batteries, either rechargeable or replaceable through surgery.
Recently, ultrasound has sparked great interest in the PNS field for powering of implants. Ultrasound is interesting for biomedical applications because the waves propagate through tissue with little scattering. Additionally, these waves can be highly focused with beamforming techniques.
By manipulating the phase and amplitude of each transducer in an array, the resulting ultrasound beam can be steered electronically without physically moving the transducer. This allows the beam to be directed towards a specific target within the body. These advantages make ultrasound a compelling alternative to inductive powering, which requires close proximity and stable alignment of the source and the implant.
A touch of ultrasound for stimulation
Not only for powering, ultrasound is also being considered for therapeutic reasons. Even though electricity is still the primary modality for PNS interaction now, ultrasound is receiving increasing attention for nerve stimulation. It is less invasive, and the ability to direct ultrasound energy to one focal point may allow more precise stimulation than electrical stimulation.
The idea is based on the concept of mid-air haptics, in which ultrasonic pressure waves at frequencies below 500Hz induce a sense of touch in the air. Imec is investigating how this principle can be used to stimulate nerves such as the vagus nerve.
Provided by IMEC
Leave a Reply