Making changes to the molecular messengers that create proteins might offer flexible therapies for cancer, pain or high cholesterol, in addition to genetic disorder
Thorsten Stafforst found his big break at the worst possible time. In 2012, his team at the University of Tübingen in Germany discovered that by linking enzymes to engineered strands of RNA, they could change the sequences of messenger RNA molecules in cells. In essence, they could rewrite the genome’s instructions en route to making proteins.
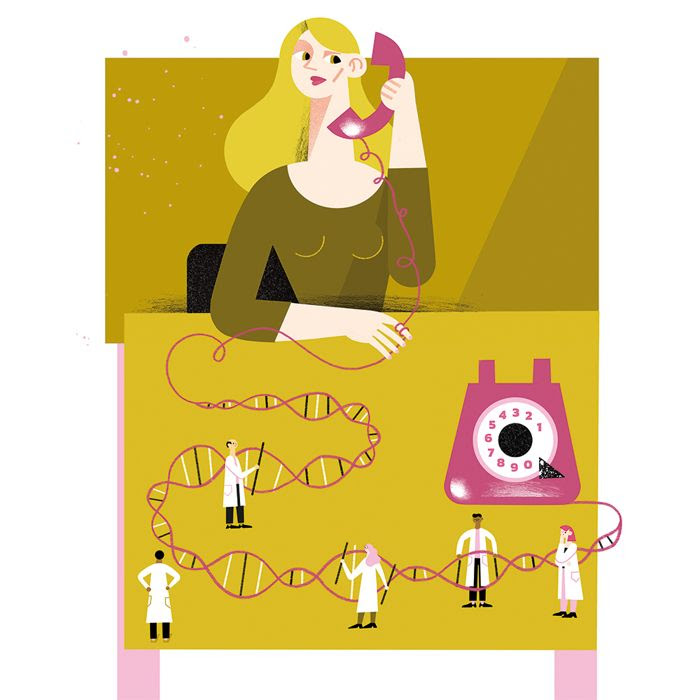
The process could theoretically serve to treat numerous diseases, both ones with genetic underpinnings and those that would benefit from a change in the amount or type of a protein being produced. But Stafforst had a lot of trouble getting the discovery published — it was simply not interesting any more. His finding1 was overshadowed by the discovery a few months earlier that the DNA-editing tool CRISPR–Cas9 could be used to permanently alter the genome.
Since then, CRISPR has become a fixture in the laboratory and has spawned a number of companies aimed at using the technology to develop drugs and treatments. With CRISPR sucking up all the attention, Stafforst says, people reacted to his paper with indifference. They asked, “Why do we need this when there’s DNA editing?”
But CRISPR editing — at least as a therapeutic technique in people — has turned out to be more difficult than initially thought. Researchers have documented ways that Cas9, one of the enzymes used in CRISPR gene editing, could trigger immune responses, or cause accidental changes to the genome that would be permanent. RNA editing, by contrast, could allow clinicians to make temporary fixes that eliminate mutations in proteins, halt their production or change the way that they work in specific organs and tissues. Because cells quickly degrade unused RNAs, any errors introduced by a therapy would be washed out, rather than staying with a person forever.
Excitement over RNA editing is finally catching on. In 2019, researchers published more than 400 papers on the topic, according to data from Scopus, an abstract and citation database. A handful of start-up companies are beginning to use RNA-editing systems to develop potential treatments for everything from genetic diseases such as muscular dystrophy to temporary maladies such as acute pain. And although RNA-based drugs have had difficulty reaching the market owing to challenges in delivery and tolerance, some regulatory approvals in the past few years might help to pave the way for RNA-editing therapies.
Several hurdles remain: current technologies can alter RNA sequences in only a few limited ways, and getting the system to work as intended in the human body will prove challenging. Still, researchers hope that new technologies, such as protein engineering, and improved methods for delivering RNA to cells can help to overcome these limitations. “It really opens a world we haven’t seen before,” Stafforst says.
A role for RNA
A foundational tenet in molecular genetics — its central dogma — was that cellular machinery faithfully transcribes genetic information from a double-stranded DNA template into a single-stranded RNA messenger, which is then translated into a protein. But in the 1980s, a handful of labs noticed that some mRNA transcripts contained altered or extra letters that were not encoded in the DNA. The findings were controversial until scientists uncovered a family of enzymes called adenosine deaminases acting on RNA (ADARs). These proteins bind to RNAs and alter their sequence by changing a familiar base known as adenosine into a molecule called inosine. Although not one of the canonical RNA bases, inosine is read by the cell’s protein-translation machinery as the familiar guanosine. A handful of other RNA-editing enzymes surfaced around the same time.
Scientists have struggled over the past three decades to understand what exactly RNA editing accomplishes. The editors work only on double-stranded RNAs, which sometimes show up in the cell as regulatory elements — or as viruses. Some have speculated that the ADAR proteins evolved as a defence against viruses, but many viruses with double-stranded RNA are unaffected by the enzymes. The editing might serve a regulatory function, but most adult tissues don’t produce the high levels of the proteins required for the editing to occur.
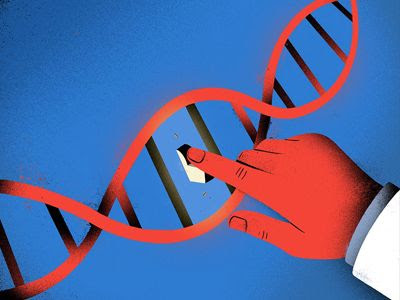
The kill-switch for CRISPR that could make gene-editing safer
Brenda Bass, a biochemist at the University of Utah in Salt Lake City, was among the first to identify ADARs in frog embryos2. She says that no one has found a specific role for the changes made to non-protein-coding RNAs, which account for the majority of edited molecules. The editing could serve to protect double-stranded RNAs from immune attack. Bass suspects that ADARs edit the double-stranded transcripts, adding inosines as a way of telling the body to leave them alone. The enzymes also seem to have a role in embryonic development: mice that lack ADAR genes die before birth or don’t live long after. The editors also seem to have some function in select tissues of adult organisms — such as the nervous system of cephalopods.
It was this activity that drew marine biologist Joshua Rosenthal to RNA editing in the early 2000s. It seems that highly intelligent cephalopods, such as squid, cuttlefish and octopuses, use RNA editing extensively to adjust genes involved in nerve-cell development and signal transmission. No other animals are known to use RNA editing in this way. Inspired by these observations, Rosenthal wondered whether it was possible to use the system to correct the messages produced by dysfunctional genes in a therapeutic setting. In 2013, his group at the University of Puerto Rico in San Juan re-engineered ADAR enzymes and attached them to guide RNAs that would bind to a specific point in an mRNA — creating a double strand. With these, they were able to edit transcripts in frog embryos, and even in human cells in culture3.
Similar to Stafforst, Rosenthal, now at the Marine Biological Laboratory in Woods Hole, Massachusetts, saw his publication mostly ignored. A similar fate, he learnt, had befallen the work of researchers at a company called Ribozyme, who in 1995 proposed ‘therapeutic editing’ of mutated RNA sequences by inserting complementary sequences into frog embryos and allowing ADARs to edit the resulting double-stranded molecule and correct the mutation4.
But in the past several years, multiple factors have converged to bring Rosenthal’s and Stafforst’s findings to the fore. Peter Beal, a chemist at the University of California, Davis, says that the 2016 publication5 of the molecular structure of ADAR bound to double-stranded RNA made the system more understandable and enabled scientists to better engineer the enzyme to enhance its delivery or make it more efficient. And in 2018, the US Food and Drug Administration (FDA) approved the first therapy using RNA interference (RNAi): a technique in which a small piece of RNA is inserted into a cell in which it binds to native mRNAs and hastens their degradation. The approval has opened the door for other therapies that involve mRNA interactions, says Gerard Platenburg, chief innovation officer of ProQR Therapeutics in Leiden, the Netherlands, which is pursuing various RNA-based therapies. “Learning from the past, and with the number of approvals picking up, the field has matured a lot,” says Platenburg.
Many see RNA editing as an important alternative to DNA editing using techniques such as CRISPR. CRISPR technology is improving, but DNA editing can cause unwanted mutations in other parts of the genome — ‘off-target effects’ — which might create new problems.
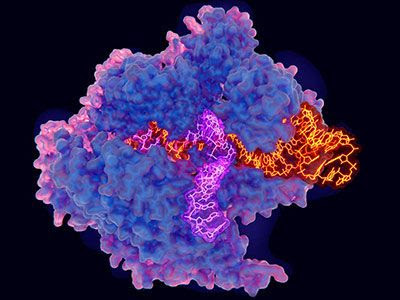
Super-precise new CRISPR tool could tackle a plethora of genetic diseases
Rosenthal expects, moreover, that RNA editing will prove useful for diseases without a genetic origin. He is currently using ADARs to edit the mRNA for a gene encoding the sodium channel Nav1.7, which controls how pain signals are transmitted to the brain. Permanently changing the Nav1.7 gene through DNA editing could eliminate the ability to feel pain and disrupt other necessary functions of the protein in the nervous system, but tuning it down through RNA editing in select tissues for a limited amount of time could help to alleviate pain without the risk of dependency or addiction associated with conventional painkillers.
Similarly, RNA editing could allow researchers to mimic genetic variants that provide a health advantage. For example, people with certain mutations in the gene PCSK9, which regulates cholesterol in the bloodstream, tend to have lower cholesterol levels, and modifying PCSK9 mRNA could confer a similar advantage without permanently disrupting the protein’s other functions. Immunologist Nina Papavasiliou of the German Cancer Research Center in Heidelberg says that RNA editing could be used to fight tumours. Some cancers hijack important cell-signalling pathways, such as those involved in cell death or proliferation. If RNA editors could be conscripted to turn off key signalling molecules temporarily, she says, “we could see the tumour die”. Then, the patient could stop the therapy, allowing the pathway to resume its normal functions.
As a treatment, RNA editing might be less likely to cause a potentially dangerous immune reaction than are CRISPR-based approaches. Unlike the DNA-editing enzyme Cas9, which comes from bacteria, ADARs are human proteins that don’t trigger an attack from the immune system. “You really don’t need heavy machinery to target RNA,” says Prashant Mali, a bioengineer at the University of California, San Diego.
In a paper published last year6, Mali and his colleagues injected guide RNAs into mice born with a genetic mutation that causes muscular dystrophy. The guide RNAs were designed to trigger production of a missing protein called dystrophin. Although the system edited only a small amount of the RNA encoding dystrophin, it restored the protein to about 5% of its normal level in the animals’ muscle tissue, an amount that has shown therapeutic potential.
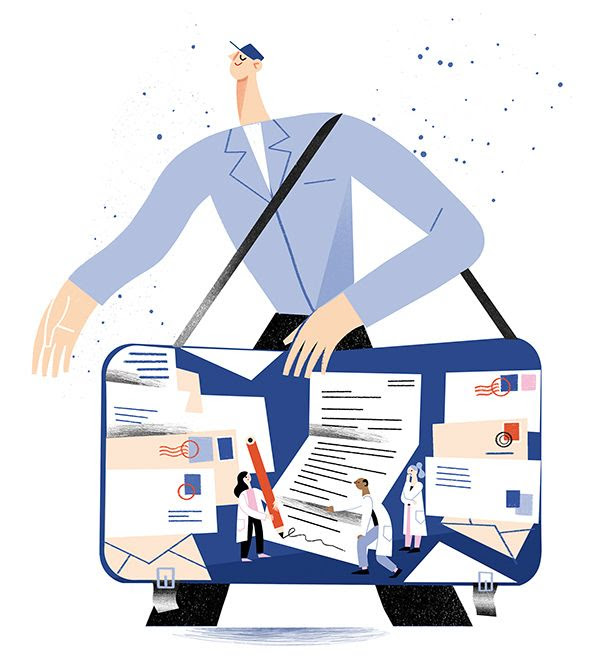
In other diseases that result from a missing or dysfunctional protein, such as some types of haemophilia, “it makes a huge difference to go from nothing to something”, Stafforst says, and it might not be necessary to edit RNA in every cell in the body. RNA editing might perform better than forms of gene therapy that would involve injecting a new gene. Mali and others say that directing native ADARs to operate on the cell’s own mRNA might provide a more natural response than introducing an external, engineered gene.
RNA-editing technology is far from perfect, however, even when it comes to laboratory applications. “It is early days,” Bass says. “There’s lots of questions.” Because ADARs are much less efficient than CRISPR, they could be less useful for making genetically modified plants and animals. “As a research tool, it’s very limiting,” says Jin Billy Li, a geneticist at Stanford University in California.
Another major disadvantage is that ADARs can make only a few kinds of change to RNA. CRISPR systems act as scissors by cutting DNA at a designated spot and removing or inserting a new sequence; ADARs are more like an overwrite function that changes letters chemically, without breaking the RNA molecule’s ‘backbone’.
Although this process is less likely to cause unintended mutations, it limits the enzymes to making specific changes — adenosine to inosine in the case of ADARs, and cytosine to uridine by a set of enzymes called APOBECs (see ‘The RNA corrections’). There are a few other possibilities. Grape plants, for instance, can change cytidines to uridines, and some tumours can change guanosines to adenosines. “Biodiversity is giving us tons of answers to these things,” Rosenthal says. “I think down the line, things like the squid are going to teach us a lot.” But he says the field is understudied — researchers don’t understand the process that drives this editing. And it remains to be seen whether a plant enzyme, for instance, could function in human cells.
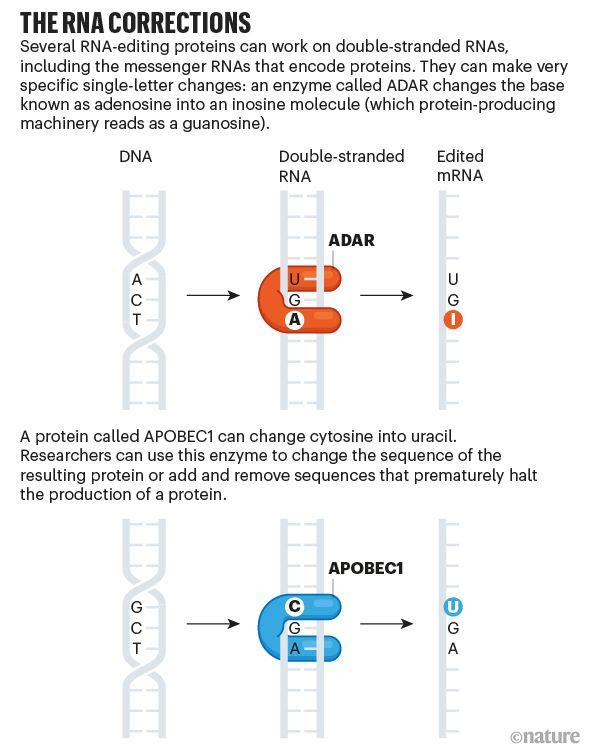
Scientists are already looking for ways to engineer new enzymes that could expand RNA-editing capabilities. “It’s quite a process where you don’t know what you’ll find,” says Omar Abudayyeh, a biological engineer at the Massachusetts Institute of Technology (MIT) in Cambridge. Working with Feng Zhang, a CRISPR pioneer at MIT, Abudayyeh and his colleagues linked an ADAR enzyme to Cas137. A bacterial enzyme similar to the CRISPR-associated protein Cas9, Cas13 cuts RNA instead of DNA. The researchers altered the sequence of the ADAR until it could convert cytidines to uridines. They then used the new system in human cells to change bases in mRNAs encoded by several genes, including APOE. One naturally occurring genetic variant of this gene is associated with Alzheimer’s disease, and editing it could switch the variant to the harmless form.
Abudayyeh and his MIT collaborator, biological engineer Jonathan Gootenberg, admit it is possible that changing the ADAR protein could cause the immune system to stop recognizing it as a natural human protein and attack cells that contain it. But they say that because these edits are small, this risk pales next to known concerns about the immune system attacking Cas13 or the virus used to deliver the editing tools into cells.
Researchers see promise in a natural process called pseudouridylation, in which a set of protein and RNA enzymes chemically modify the structure of uridines in mRNA. Unlike ADAR modifications, pseudouridylation doesn’t change the sequence of the mRNA or protein. Instead, for reasons that are not entirely clear, the process stabilizes the RNA molecule and causes the translation machinery to ignore signals instructing it to stop making protein.
The ability to turn these molecular red lights into green lights could be powerful. Yi-Tao Yu, a biochemist at the University of Rochester in New York, says that hundreds of genetic diseases are caused by DNA mutations that create incorrect stop signals in mRNAs, resulting in a shortened protein that doesn’t function normally in the body. “The list is very long,” Yu says, and includes cystic fibrosis, the eye disease Hurler’s syndrome and numerous cancers.
Despite its early stage, researchers — and biotech investors — are excited about the wide potential of RNA editing. “I got into it way before it became cool,” says Papavasiliou, who is trying to map where natural ADARs work in the body. “For many years this was a backwater, and all of a sudden there’s a company popping up every two weeks.”
Numerous start-ups and established DNA-editing firms have announced their intention to move into RNA. They include Beam Therapeutics in Boston, Massachusetts, which was co-founded by Zhang and Liu and has been developing CRISPR DNA editing as a therapy for several blood diseases. Locana, based in San Diego, is also pursuing CRISPR-based RNA editing that it hopes could treat conditions including motor-neuron disease and Huntington’s disease.
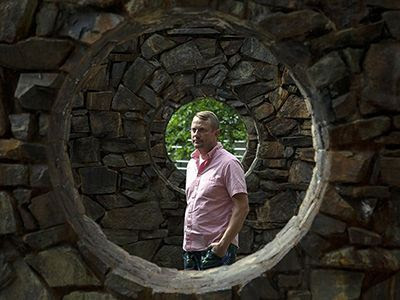
CRISPR babies: when will the world be ready?
The challenge for industry is to work out the best way to get the guide RNAs into the cell without triggering an immune reaction or causing the cell to degrade them. Beal says that this could include making strategic chemical modifications to the engineered RNAs that stabilize them, or embedding them in a nanoparticle or virus that can sneak into cells.
And although ADARs are already in human cells, the human body makes only small amounts of them in most tissues, meaning that any therapy might need to add ADARs or other enzymes to boost cells’ editing capabilities. Packing viruses with the genes that encode all the machinery needed for RNA editing might not be efficient. Many hope that it won’t be necessary.
Platenburg hopes to add RNAs and rely on the naturally occurring ADARs to help to correct the lettering of mRNAs that contribute to retinal disorders. “We use the system given to us by nature and harness it,” he says.
Researchers including Stafforst are engineering guide RNAs with chemical modifications that attract ADARs in the cell to the editing site. But some researchers worry that conscripting the natural ADARs into editing specific mRNAs could pull them away from their normal tasks and cause other health problems. Altering gene expression in one part of the body could affect other parts in unforeseen ways. In Mali’s muscular-dystrophy study, for instance, mice developed liver problems for unknown reasons. “It’s a tool in development still,” he says.
“ADAR evolved to allow the body to modify bases in a very targeted fashion,” says Nessan Bermingham, chief executive and a co-founder with Rosenthal and others of biotechnology company Korro Bio in Cambridge, Massachusetts. Bermingham is optimistic about the prospects of RNA editing, but cautious not to get ahead of the biology. “We have a lot of work to do as we start to mature these techniques,” he says. “We’re not leaving anything off the table, but we have to recognize certain limitations.”
Leave a Reply